MENU
AE | USD
-
-
-
-
- Forum Labo 2025
- Advanced Therapies Week (ATW) 2025
- SLAS Europe 2025
- Bioprocessing Summit Europe 2025
- Medlab Middle East 2025
- SLAS International 2025
- Biologics World Nordics 2025
- ASIA LABEX: The Lab Show 2025
- BioProcess International Europe 2025
- ISEV 2025
- Future Labs Live 2025
- Cell 2025
- ASIA LABEX: The Lab Show 2025
- Stem Cell Community Day 2025
-
-
-
-
- Forum Labo 2025
- Advanced Therapies Week (ATW) 2025
- SLAS Europe 2025
- Bioprocessing Summit Europe 2025
- Medlab Middle East 2025
- SLAS International 2025
- Biologics World Nordics 2025
- ASIA LABEX: The Lab Show 2025
- BioProcess International Europe 2025
- ISEV 2025
- Future Labs Live 2025
- Cell 2025
- ASIA LABEX: The Lab Show 2025
- Stem Cell Community Day 2025
AE | USD
-
- Benchtop Centrifuges
- Floor-Standing Centrifuges
- Refrigerated Centrifuges
- Microcentrifuges
- Multipurpose Centrifuges
- High-Speed Centrifuges
- Ultracentrifuges
- Concentrator
- High-Speed and Ultracentrifuge Consumables
- Centrifuge Tubes
- Centrifuge Plates
- Device Management Software
- Sample and Information Management
-
- All Pipettes, Dispensers & Automated Liquid Handlers
- Mechanical Pipettes
- Electronic Pipettes
- Multi-Channel Pipettes
- Positive Displacement Pipettes & Dispensers
- Automated Pipetting
- Bottle-Top Dispensers
- Pipette Controllers
- Pipette Tips
- Automation Consumables
- Dispenser & Pipette Accessories
- Automation Accessories
- Dispenser & Pipette Services
You are about to leave this site.
Please be aware that your current cart is not saved yet and cannot be restored on the new site nor when you come back. If you want to save your cart please login in into your account.
No results found
Search Suggestions
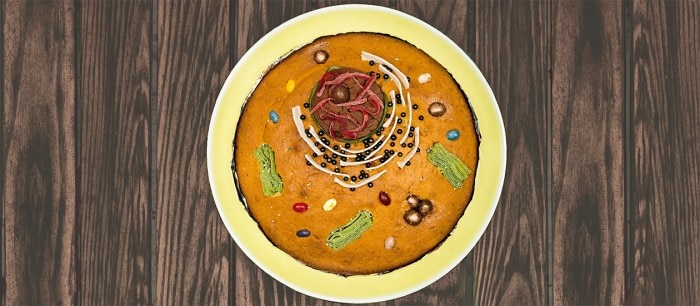
Cho Turns 60 – The Hamster That Keeps on Going
Beyond Science
- Cell types
- Essay
- Inspiring Science
2017 marks the 60th anniversary of a true mainstay of protein research and bioprocessing. First established in 1957, the Chinese Hamster Ovary (CHO) cell line was one of the first stable mammalian cell lines developed, and continues to be valuable tool in research and industry.
Pioneering cell culture
The 1950s was a real golden age of cell culture. Famously, 1951 saw George Gey develop the human HeLa cell line from cervical cancer tissue1 . Soon after, Theodore Puck, another pioneer in the emerging field of cell culture, conducted some of his most groundbreaking research. Based at the University of Colorado Medical Center in Denver, Puck successfully cloned the HeLa cell in 1955 and worked on the research that identified humans have 46 chromosomes rather than 48 as previously thought2 .
And then, somebody gave Puck a hamster...
Puck established the CHO cell line by isolating epithelial cells from the ovaries of a Chinese hamster (Cricetulus griseus). Already used as a laboratory animal in previous decades, these hamsters had been a good animal model in, for example, radiation studies. Upon culture in vitro, the cells spontaneously immortalized3 , paving the way for unlimited expansion and use at the largest scales.
The prolific cell
In the decades that followed, the CHO cell became a familiar ‘face’ in biolabs all over the world. Publications throughout the 1960s and ‘70s report the use of CHO cells to study DNA damage, effects of radiation, viral infection and propagation, and effects of chemicals on cell proliferation among many others.
Following the widespread adoption of CHO cells, Puck was awarded the 1973 Louisa Gross Horwitz Prize from Columbia University together with two other greats in the field of cell culture: Renato Dulbecco and Harry Eagle (whose names will be familiar to those working in cell culture labs).
Highlighting Puck’s achievements, the awards committee called him “the first to demonstrate that individual animal cells in culture can give rise to colonies.” It also stated that CHO cell culture “has proven a pivotal method in the development of animal cell biology”4 .
One reason for the enduring success of the CHO cell line is the fact that it is highly suitable for transfection studies. The combination of good genome stability and high rates of vector incorporation means that it is the ideal tool for producing recombinant proteins. After translation, proteins often undergo post-translational modifications (PTMs), which affect their efficacy and immunogenicity. These mammalian cells tend to perform the same PTMs as human cells, giving CHO cells an advantage over using bacterial production3,5 .
The selective advantage
From the original CHO cell line, researchers developed several variants to improve its performance. One of the most significant developments came in the 1980s with the introduction of two variant cell lines that do not express dihydrofolate reductase (DHFR): CHO-DUKX and CHO-DG446.
DHFR is an enzyme involved in the synthesis of certain amino acids; absence of DHFR activity means that cells become reliant on glycine, hypoxanthine and thymidine (GHT) for survival. This characteristic can be exploited in search of an efficient method for producing recombinant proteins.
Researchers created vectors that contain both the gene of interest and the DHFR gene (as a selectable marker). This means that cells that take up the vector are no longer reliant on supplemented medium, and if GHT is withdrawn, only the cells that contain the gene of interest will proliferate. This approach helped to create a more efficient protein production process, extending the usefulness and lifetime of CHO cells as a tool in cell culture into the modern age.
Workhorse of bioprocessing
Driven by a high demand for recombinant proteins at minimal cost, researchers set out to improve protein production in CHO cells through optimized culture media, better selection strategies, and many other factors. These optimizations have led to a 20-fold increase in yield since the 1980s. As a result, industrial protein production processes can achieve titers of 1–5 g/L, minimizing costs and making the production of a larger range of proteins more economically viable6 .
Developments in the composition of cell culture media have also resulted in serum-free chemically-defined media suitable for CHO cells. Use of these media has opened the possibility for xeno-free CHO protein production, which, combined with low risks of viral contamination, improves the chance of regulatory approval3. Highlighting the success of this cell line, more than 50% of the biopharmaceuticals approved in the US and Europe between 2006 and 2010 were produced in CHO cells7 .
Happy birthday CHO
In the age of cell culture, CHO was there from the beginning and is still going strong at 60. With an established history of regulatory approval for recombinant protein expression8 , protein yields in the gram-per-liter range, and applications in many medicines today, CHO shows no signs of slowing down. So... let’s take a moment to celebrate these amazing little protein factories. Hip, hip...
The 1950s was a real golden age of cell culture. Famously, 1951 saw George Gey develop the human HeLa cell line from cervical cancer tissue1 . Soon after, Theodore Puck, another pioneer in the emerging field of cell culture, conducted some of his most groundbreaking research. Based at the University of Colorado Medical Center in Denver, Puck successfully cloned the HeLa cell in 1955 and worked on the research that identified humans have 46 chromosomes rather than 48 as previously thought2 .
And then, somebody gave Puck a hamster...
Puck established the CHO cell line by isolating epithelial cells from the ovaries of a Chinese hamster (Cricetulus griseus). Already used as a laboratory animal in previous decades, these hamsters had been a good animal model in, for example, radiation studies. Upon culture in vitro, the cells spontaneously immortalized3 , paving the way for unlimited expansion and use at the largest scales.
The prolific cell
In the decades that followed, the CHO cell became a familiar ‘face’ in biolabs all over the world. Publications throughout the 1960s and ‘70s report the use of CHO cells to study DNA damage, effects of radiation, viral infection and propagation, and effects of chemicals on cell proliferation among many others.
Following the widespread adoption of CHO cells, Puck was awarded the 1973 Louisa Gross Horwitz Prize from Columbia University together with two other greats in the field of cell culture: Renato Dulbecco and Harry Eagle (whose names will be familiar to those working in cell culture labs).
Highlighting Puck’s achievements, the awards committee called him “the first to demonstrate that individual animal cells in culture can give rise to colonies.” It also stated that CHO cell culture “has proven a pivotal method in the development of animal cell biology”4 .
One reason for the enduring success of the CHO cell line is the fact that it is highly suitable for transfection studies. The combination of good genome stability and high rates of vector incorporation means that it is the ideal tool for producing recombinant proteins. After translation, proteins often undergo post-translational modifications (PTMs), which affect their efficacy and immunogenicity. These mammalian cells tend to perform the same PTMs as human cells, giving CHO cells an advantage over using bacterial production3,5 .
The selective advantage
From the original CHO cell line, researchers developed several variants to improve its performance. One of the most significant developments came in the 1980s with the introduction of two variant cell lines that do not express dihydrofolate reductase (DHFR): CHO-DUKX and CHO-DG446.
DHFR is an enzyme involved in the synthesis of certain amino acids; absence of DHFR activity means that cells become reliant on glycine, hypoxanthine and thymidine (GHT) for survival. This characteristic can be exploited in search of an efficient method for producing recombinant proteins.
Researchers created vectors that contain both the gene of interest and the DHFR gene (as a selectable marker). This means that cells that take up the vector are no longer reliant on supplemented medium, and if GHT is withdrawn, only the cells that contain the gene of interest will proliferate. This approach helped to create a more efficient protein production process, extending the usefulness and lifetime of CHO cells as a tool in cell culture into the modern age.
Workhorse of bioprocessing
Driven by a high demand for recombinant proteins at minimal cost, researchers set out to improve protein production in CHO cells through optimized culture media, better selection strategies, and many other factors. These optimizations have led to a 20-fold increase in yield since the 1980s. As a result, industrial protein production processes can achieve titers of 1–5 g/L, minimizing costs and making the production of a larger range of proteins more economically viable6 .
Developments in the composition of cell culture media have also resulted in serum-free chemically-defined media suitable for CHO cells. Use of these media has opened the possibility for xeno-free CHO protein production, which, combined with low risks of viral contamination, improves the chance of regulatory approval3. Highlighting the success of this cell line, more than 50% of the biopharmaceuticals approved in the US and Europe between 2006 and 2010 were produced in CHO cells7 .
Happy birthday CHO
In the age of cell culture, CHO was there from the beginning and is still going strong at 60. With an established history of regulatory approval for recombinant protein expression8 , protein yields in the gram-per-liter range, and applications in many medicines today, CHO shows no signs of slowing down. So... let’s take a moment to celebrate these amazing little protein factories. Hip, hip...
Read more
Read less
References
1. Lucey BP et al. Henrietta Lacks, HeLa Cells, and Cell Culture Contamination. Archives of Pathology & Laboratory Medicine 2009;133(9): 1463–1467.
2. Rawley JD. Theodore T. Puck (September 24, 1916–November 6, 2005). American Journal Human Genetics 2006;78(3): 365–366.
3. Fisher S et al. The art of CHO cell engineering: A comprehensive retrospect and future perspectives. Biotechnology Advances 2015;33(8): 1878–96.
4. University Record. Horwitz prize. University Record (Columbia University) 1973;1(4): 1–2.
5. Kim JY et al. CHO cells in biotechnology for production of recombinant proteins: current state and further potential. Applied Microbiology and Biotechnology 2012;93(3): 917–930.
6. Wuest DM et al. Genomics in mammalian cell culture bioprocessing. Biotechnological Advances 2012;30(3): 629–638.
7. Walsh G. Biopharmaceutical benchmarks 2010. Nature Biotechnology 2010;28(9): 917–924.
8. Xu X et al. The Genomic Sequence of the Chinese Hamster Ovary (CHO) K1 cell line. Nature Biotechnology 2012;29(8): 735–741.
1. Lucey BP et al. Henrietta Lacks, HeLa Cells, and Cell Culture Contamination. Archives of Pathology & Laboratory Medicine 2009;133(9): 1463–1467.
2. Rawley JD. Theodore T. Puck (September 24, 1916–November 6, 2005). American Journal Human Genetics 2006;78(3): 365–366.
3. Fisher S et al. The art of CHO cell engineering: A comprehensive retrospect and future perspectives. Biotechnology Advances 2015;33(8): 1878–96.
4. University Record. Horwitz prize. University Record (Columbia University) 1973;1(4): 1–2.
5. Kim JY et al. CHO cells in biotechnology for production of recombinant proteins: current state and further potential. Applied Microbiology and Biotechnology 2012;93(3): 917–930.
6. Wuest DM et al. Genomics in mammalian cell culture bioprocessing. Biotechnological Advances 2012;30(3): 629–638.
7. Walsh G. Biopharmaceutical benchmarks 2010. Nature Biotechnology 2010;28(9): 917–924.
8. Xu X et al. The Genomic Sequence of the Chinese Hamster Ovary (CHO) K1 cell line. Nature Biotechnology 2012;29(8): 735–741.
Read more
Read less