MENU
US | USD
-
-
-
-
- Forum Labo 2025
- Advanced Therapies Week (ATW) 2025
- SLAS Europe 2025
- Bioprocessing Summit Europe 2025
- Medlab Middle East 2025
- SLAS International 2025
- Biologics World Nordics 2025
- ASIA LABEX: The Lab Show 2025
- BioProcess International Europe 2025
- ISEV 2025
- Future Labs Live 2025
- Cell 2025
- ASIA LABEX: The Lab Show 2025
-
-
-
-
- Forum Labo 2025
- Advanced Therapies Week (ATW) 2025
- SLAS Europe 2025
- Bioprocessing Summit Europe 2025
- Medlab Middle East 2025
- SLAS International 2025
- Biologics World Nordics 2025
- ASIA LABEX: The Lab Show 2025
- BioProcess International Europe 2025
- ISEV 2025
- Future Labs Live 2025
- Cell 2025
- ASIA LABEX: The Lab Show 2025
US | USD
-
- Benchtop Centrifuges
- Floor-Standing Centrifuges
- Refrigerated Centrifuges
- Microcentrifuges
- Multipurpose Centrifuges
- High-Speed Centrifuges
- Ultracentrifuges
- Concentrator
- High-Speed and Ultracentrifuge Consumables
- Centrifuge Tubes
- Centrifuge Plates
- Device Management Software
- Sample and Information Management
-
- All Pipettes, Dispensers & Automated Liquid Handlers
- Mechanical Pipettes
- Electronic Pipettes
- Multi-Channel Pipettes
- Positive Displacement Pipettes & Dispensers
- Automated Pipetting
- Bottle-Top Dispensers
- Pipette Controllers
- Pipette Tips
- Automation Consumables
- Dispenser & Pipette Accessories
- Automation Accessories
- Dispenser & Pipette Services
No results found
Search Suggestions
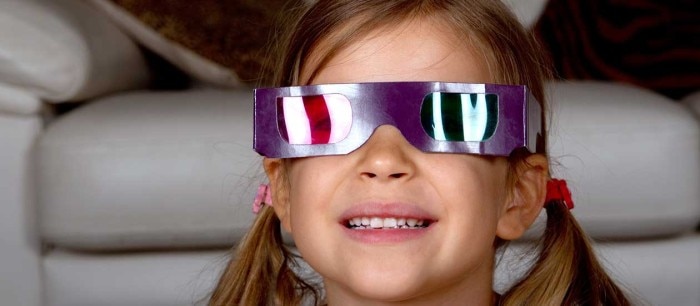
How do cells cultured in 2D and 3D differ? A dimension shift
Lab Academy
- Cell Biology
- Cell Culture
- Stem Cells
- Lab Routine
- Reproducibility
- Cell Culture Consumables
- Essay
This article appeared first in “ Inside Cell Culture ”, the monthly newsletter for cell culture professionals
Read more
Read less
In this article in a series of cell culture articles , we look at one of the biggest developments in cell culture approaches; the transition from 2D to 3D.
Read more
Read less
2D and 3D cell cultures: what’s the difference?
2D cell culture remains the most common method of growing cells. Cells are seeded on flat surfaces (typically Petri dishes, flasks or plates), where supporting media encourages proliferation and cells grow in a monolayer (Figure 1). For many years, 2D cell culture has offered scientists a simple and low-cost method to maintain cells and perform experiments; such as testing cellular responses to drug candidates. However, in recent years, 3D cell culture techniques have caused quite a stir in the scientific community.
In contrast to 2D cell culture, cells in 3D cell culture can interact with their surroundings in all three dimensions. In 3D culture, cells often grow to form spheroids - cells that arrange themselves during proliferation into sphere-like formations (Figure 1). 3D cell cultures can be prepared using a support known as a scaffold which allows growth in all directions. Examples of scaffolds include hydrogels, polymeric hard materials, and hydrophilic glass fibre1. In scaffold-based 3D cultures using an extracellular matrix (ECM), cells are embedded into the matrix whereas hard polymer scaffolds can provide the physical support of a specialized tissue such as skin, tendons, or bones (Figure 1).
Scaffold-free approaches also exist and these rely on the self-aggregation of cells. One of the most well-known scaffold-free approaches is the hanging drop method; a technique that can be used both with and without specialist plates1,2 . In this method, cells are encouraged to naturally form cellular aggregates, facilitated by gravitational and surface tension forces. In addition, bioreactors are increasingly used for large-scale cell culture. Examples of these include general bioreactors that are independent of a CO2 incubator, to alternative bioreactors, such as orbital shakers, spinner flasks, and rotating wall vessels3. All of which employ motion to prevent cells from adhering to surfaces, keeping cells and spheroids in solution (Figure 1).
2D and 3D cell cultures offer scientists a model platform for a wide range of applications. But, how do we know which one to choose?
Read more
Read less
2D vs 3D cell culture: advantages and limitations
2D cell culture approaches are well-established. This translates to straight-forward protocols and ready-to-use models that are typically easy to analyze. Moreover, there is a wealth of comparative literature available that lends itself to framing experimental results in the context of the wider field of study.
However, 2D cell cultures harbor several limitations (Table 1). Most importantly, 2D cultured cells are not reflective of the natural structure of tissues; and as such, cell-cell and cell-environment interactions are not fully represented. This has driven the development of alternative models that better mimic the physiology of biological tissues – such as 3D culture systems.
Table 1. A comparison between 2D and 3D cell cultures. Table reproduced from Kapałczyńska et al. (2018)Cells4
Read more
Read less
Characteristic | 2D cell culture | 3D cell culture |
Cell morphology and polarity (Figure 2) | Flat, stretched, partially polarised cells in monolayer | Morphology mirrors natural tissue structure and cell polarization |
Representativity of in vivo conditions | Do not mimic natural structure | 3D structure is representative of tissue organization |
Time to culture formation | Minutes to hours | Hours to days |
Cell-cell and cell-environment interactions | Limited ability to interact with each other and the extracellular environment | Interactions mimic in vivo interactions |
Access to essential compounds | Unlimited access to essential compounds (e.g., nutrients and metabolites) | Variable access to essential compounds as gradients are a consequence of the 3D structure |
Gene and protein expression | Expression patterns are often different from in vivo models | Expression pattens can be similar to in vivo models |
Cost of maintaining culture | Reagents and consumables for 2D culture are usually widely available and inexpensive | 3D cell culturing is expensive with fewer commercially available components. |
Read more
Read less
The most important advantage of 3D cultures is that they serve as a better simulation of what is happening in vivo. By the creation of complex systems, linked together by microfluidic channels, 3D cultures can emulate how different cell types interact. Additionally, the 3D structure provides variable access to essential compounds – such as oxygen, nutrients, and metabolites – in a way that reflects an in vivo system. As a consequence, 3D models provide a better and more accurate insight into topics such as normal physiological function, disease progression, and drug toxicity and potency testing.
Read more
Read less
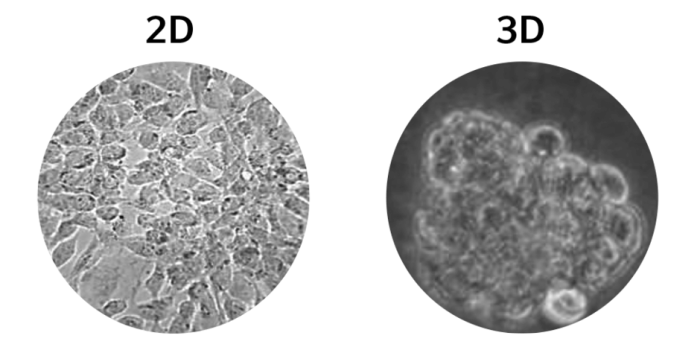
Figure 2. (Left) 2D cell culture of Caco-2 cells, (right) 3D cell culture of Caco-2 cells5.
There are, however, some significant challenges associated with using 3D cell culture approaches. First and foremost, protocols are generally not defined and consequently, require significant time and monetary input. The lack of established methodology also translates to significant variability between labs and a general lack of reproducibility. Nevertheless, with innovative companies developing increasingly high-throughput, accessible, and reproducible 3D model platforms, it seems likely that 3D cell culture will quickly become as popular as 2D cell culture approaches.
Although 3D cell culture has been around from as early as 1870, the last 5 to 10 years6 have seen significant investment and correspondingly, considerable advances - especially in the fields of organoids7 , organ-on-a-chip (tissue chips)8 , and 3D-bioprinting technologies9 .
Organoids can be defined as self-assembled 3D tissue structures that are derived from primary tissues or stem cells while an organ-on-a-chip is a tiny microfluidic device that contains channels lined by living cells and tissues.
3D-bioprinting is a technique used in 3D culture in which computer designed structures can be created from multiple cell types, biomaterials, and biomolecules. Bioprinting can be used to generate both organoids and organ-on-a-chip devices, which have different advantages depending on scientific objective. For instance, tissue chips provide fluid flow and relevant mechanical cues and reconstitute tissue-tissue interfaces. Additionally, since chip technology supports flow, it is highly amenable to long-term co-culture (e.g. with commensal microbes) and enables physiologically relevant recruitment of circulating immune cells. On the other hand, organoids provide greater insight into how normal organs are built and maintained.
These cutting-edge technologies have the potential to transform our understanding of human biology and disease pathology as well as revolutionize therapeutic development. For example, both organoids and organ-on-a-chip technologies offer new platforms to speed up the therapeutic screening process since they closely mimic human tissues and can be extremely high-throughput. Moreover, 3D bioprinting technology is already capable of printing organoids at high speeds in 96-well plate formats, streamlining the process further. In turn, the implementation of these technologies into the drug discovery and development pipeline could not only help reduce the high cost of getting a drug to market, but also reduce our reliance on animal models.
3D cell culture: advances and applications
Although 3D cell culture has been around from as early as 1870, the last 5 to 10 years6 have seen significant investment and correspondingly, considerable advances - especially in the fields of organoids7 , organ-on-a-chip (tissue chips)8 , and 3D-bioprinting technologies9 .
Organoids can be defined as self-assembled 3D tissue structures that are derived from primary tissues or stem cells while an organ-on-a-chip is a tiny microfluidic device that contains channels lined by living cells and tissues.
3D-bioprinting is a technique used in 3D culture in which computer designed structures can be created from multiple cell types, biomaterials, and biomolecules. Bioprinting can be used to generate both organoids and organ-on-a-chip devices, which have different advantages depending on scientific objective. For instance, tissue chips provide fluid flow and relevant mechanical cues and reconstitute tissue-tissue interfaces. Additionally, since chip technology supports flow, it is highly amenable to long-term co-culture (e.g. with commensal microbes) and enables physiologically relevant recruitment of circulating immune cells. On the other hand, organoids provide greater insight into how normal organs are built and maintained.
These cutting-edge technologies have the potential to transform our understanding of human biology and disease pathology as well as revolutionize therapeutic development. For example, both organoids and organ-on-a-chip technologies offer new platforms to speed up the therapeutic screening process since they closely mimic human tissues and can be extremely high-throughput. Moreover, 3D bioprinting technology is already capable of printing organoids at high speeds in 96-well plate formats, streamlining the process further. In turn, the implementation of these technologies into the drug discovery and development pipeline could not only help reduce the high cost of getting a drug to market, but also reduce our reliance on animal models.
Read more
Read less
References
1. Jensen, C. & Teng, Y. Is It Time to Start Transitioning From 2D to 3D Cell Culture? Front. Mol. Biosci. 33 (2020).
2. Foty, R. A simple hanging drop cell culture protocol for generation of 3D spheroids. Journal of visualized experiments : JoVE ,51 2720. (2011) doi:10.3791/2720.
3. Ahmed, S., Chauhan, V.M., Ghaemmaghami, A.M. et al. New generation of bioreactors that advance extracellular matrix modelling and tissue engineering. Biotechnol Lett 41, 1–25 (2019) doi:10.1007/s10529.
4. Kapałczyńska, M. et al. 2D and 3D cell cultures – a comparison of different types of cancer cell cultures. Arch. Med. Sci. 14, 910 (2018).
5. Wu, Zhipan & Guan, Rongfa & Tao, Miao & Lyu, Fei & Cao, Guozhou & Liu, Mingqi & Gao, Jianguo. Assessment of the toxicity and inflammatory effects of differentsized zinc oxide nanoparticles in 2D and 3D cell cultures. RSC Adv.. 7. 12437- 12445. (2017) doi: 10.1039/C6RA27334C.
6. Jedrzejczak-Silicka, M. History of Cell Culture. New Insights into Cell Cult. Technol. (2017) doi:10.5772/66905.
7. Shankaran, A., Prasad, K., Chaudhari, S. et al. Advances in development and application of human organoids. 3 Biotech 11, 257 (2021) doi: 10.1007/s13205.
8. Wu, Q., Liu, J., Wang, X. et al. Organ-on-a-chip: recent breakthroughs and future prospects. BioMed Eng OnLine 19, 9 (2020) doi: 10.1186/s12938.
9. Dey, M., Ozbolat, I.T. 3D bioprinting of cells, tissues and organs. Sci Rep 10, 14023 (2020).
Read more
Read less