MENU
ZA | ZAR
ZA | ZAR
You are about to leave this site.
Please be aware that your current cart is not saved yet and cannot be restored on the new site nor when you come back. If you want to save your cart please login in into your account.
myEppendorf
No results found
Search Suggestions
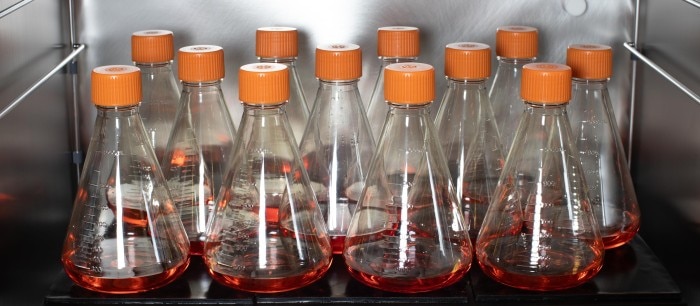
Top tips for maximizing protein production in mammalian cells
Lab Academy
- Pharma
- Cell Biology
- Cell Culture
- Efficiency
- Reproducibility
- Scalability
- Essay
This article was published first in " Inside Cell Culture " , the monthly newsletter for cell culture professionals. Find more interesting articles about CO2 incubators/shakers on our page " FAQs and material on CO2 incubators ".
Contents
Contents
Read more
Read less
Introduction - Protein production in mammalian cells
Protein production is a fundamental part of scientific research and industrial processes, helping to unravel the role of proteins in cellular pathways, and also being crucial for the development of therapeutics, vaccines, and diagnostic reagents. Despite the importance of protein production, attaining high yields can often be a challenge that hinders both research progress and industrial applications, due to the metabolic burden that recombinant protein expression places on host cells.Most complex recombinant proteins for biopharmaceutical production and therapeutic use are produced in mammalian cell lines, such as Chinese Hamster Ovary (CHO) or Human Embryonic Kidney 293 (HEK293) cells, since they can produce large quantities of proteins and can generate human-like post-translational modifications (PTMs), which are essential for protein folding and biological activity.
While substantial progress has been made in mammalian cell-based expression systems, the production of complex novel protein therapeutics, such as viral antigens, vaccines, and bi- and tri-specific monoclonal antibodies, often remains a significant challenge [1].
In response to this, efforts have been directed toward optimizing gene sequences, expression vectors, cell culture media, and culture conditions to facilitate the increased production of functional recombinant proteins. In this article, we discuss how these parameters can be optimized to increase protein production over prolonged periods while maintaining cellular integrity and stability and minimizing metabolic burden.
Read more
Read less
Vector optimization
Vector optimization is crucial for enhancing protein production, requiring the careful consideration of not only gene coding sequences, but also noncoding DNA elements like the promoter and fusion tags. These elements are essential for fine-tuning protein production. While it may be tempting to maximize all of these parameters, this can lead to lower protein yields due to inadequate cell resources. And this can result in a pool of aggregated, misfolded, or insoluble proteins.As such, achieving optimal protein expression requires careful vector optimization for maximum production of biologically functional recombinant proteins. While there's no onesize-fits-all formula for the perfect expression vector, various strategies can be employed to optimize protein production.
Read more
Read less
1. Codon usage
In recombinant protein production, the rate, yield, and final quality of the product are intricately tied to the codon usage of the target gene and the host expression vector. The use of specific codons can greatly influence mRNA stability and translation efficiency, improving the overall speed, quantity, and quality of the resulting product. Therefore, optimizing the choice of codons to ensure better compatibility between the target gene and the host expression vector can significantly enhance production outcomes [2-4].For example, across the human genome, genes with a guanine (G) or cytosine (C) codon at the third base position are associated with increased mRNA stability and abundance [5]. Therefore, increasing the GC content at the third codon position in the expression of human recombinant proteins can effectively enhance protein yields. This has been shown in CHO cells expressing the recombinant human interferon beta (rhIFN-β) gene, where introducing a G or C at each third base position increased the gene expression level by 2.8-fold [3].
However, some studies have also shown that synonymous codon changes may negatively affect product quality, reducing protein conformation and stability, changing sites of PTMs, and altering protein folding and function [6]. Therefore, although the strategic modification of codons can be an excellent tool for alleviating translation bottlenecks and enhancing protein production, it’s also important to understand the potential impact of these changes on the final product.
2. Promoter selection
Promoters play an essential role in regulating gene expression and are crucial for producing very high and stable transgene expression in mammalian cell cultures. Strong viral-derived promoters, such as human cytomegalovirus (hCMV) or simian virus 40 (SV40), are frequently used for the constitutive expression of recombinant proteins in mammalian cells, for both commercial and research purposes [7].Although these promoters achieve high expression levels, the sustained overexpression of recombinant proteins can elicit a cellular stress response similar to heat shock or oxidative stress. This can lead to reduced protein expression in the cell to conserve energy and resources. Moreover, viral-derived promoters, including hCMV and SV40, are susceptible to epigenetic silencing, further impeding the long-term production of recombinant proteins [8].
Current research is exploring the use of natural, endogenous promoters in recombinant protein expression systems as an alternative to viral-derived promoters. These are promoter sequences that are naturally expressed in the host cell. When fused to the target gene, strong endogenous promoters can induce high levels of recombinant protein expression. Their compatibility with host regulatory elements helps to avoid epigenetic silencing and cellular stress responses, while improving translation efficiency [8,9]. This shift in promoter choice represents a promising opportunity for optimizing protein production in mammalian cell cultures.
4. Fusion tags
Fusion tags are small proteins or peptides that are fused onto recombinant proteins to improve their solubility, folding, and purification, all of which are essential for achieving high product yields [10, 11]. To optimize recombinant protein production, fusion tags fall into two primary categories: affinity tags and solubility tags.Affinity tags aid protein purification, whereas solubility tags play a critical role in facilitating protein folding and maintaining solubility. Therefore, solubility tags are particularly useful for recombinant proteins with complex PTMs, or larger recombinant proteins that are prone to aggregation. Widely used fusion tags in mammalian cell lines include polyhistidine tags (Histags) for protein purification [12], and maltose-binding protein (MBP), which has dual affinity and solubility functions [13].
However, no single tag is universally successful, and the choice of each tag will depend on several factors such as the required size, location, and composition of the tag, protein production levels, and the host organism used. To further improve their performance, fusion tags are often fused with more than one tag. Using affinity and solubility-enhancing tags together can allow researchers to solve expression, solubility, and purification problems.
5. Chaperones
Protein folding is a major bottleneck in protein expression systems, yet is essential for maintaining the biological function of the recombinant protein. Therefore, the co-expression of chaperones, which assist in protein folding, can ensure that the recombinant protein maintains its native conformation and prevents aggregation. This is particularly valuable when optimizing the production of complex or challenging-to-express proteins.A proven strategy for optimizing protein yields involves co-expressing endoplasmic reticulum (ER) chaperones such as the heat shock protein 70 family (HSP70) and protein disulfide isomerase (PDI). For example, the overexpression of ER-related chaperones like binding immunoglobulin protein (BiP) and PDI in CHO cells has been shown to decrease aggregates, improve protein folding and assembly, and increase the titer of difficult-to-express recombinant proteins and monoclonal antibodies [14-16].
However, it's important to note that the effectiveness of these strategies can be protein-specific, and different proteins may benefit to varying extents from the overexpression of different chaperones.
Read more
Read less
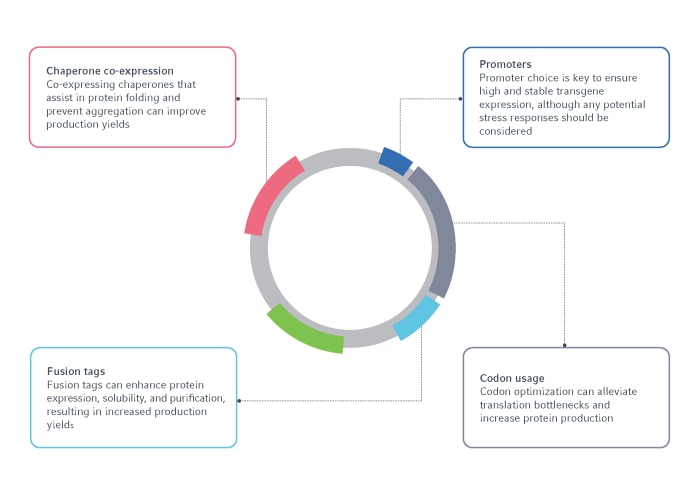
Figure 1: A summary of the vector elements requiring optimization for recombinant protein production.
Cell culture optimization
In addition to the vectors used, cell culture media and process parameters also play a crucial role in recombinant protein production. By providing the cells with essential nutrients and the proper environment for cell growth and productivity, high cell densities and efficient protein synthesis can be achieved. This ultimately improves the quality and yield of recombinant proteins in mammalian cell production systems.Read more
Read less
Media composition
For years, mammalian cell growth has relied on serum-supplemented media. However, recent advancements have propelled the evolution of serum-free, chemically defined media (CDM) for CHO and other mammalian cell lines. The use of custom media enables scientists to have a deeper understanding of the impact of key media components on cell health and productivity.Therefore, custom CDM can be advantageous in facilitating efficient process optimization, troubleshooting any decreases in cell growth or protein yields and minimizing variabilities in production [17].
Typically, CDM comprises 50-100 components [18], including carbohydrates, amino acids, vitamins, trace metals, salts, lipids, and buffers. These components need to be present at optimal concentrations for maintaining cell-specific growth, productivity, and product quality. However, media development generally focuses on optimizing concentrations of the key components, such as glucose as a primary source of energy supply, and amino acids for cell density and protein titer [19].
Read more
Read less
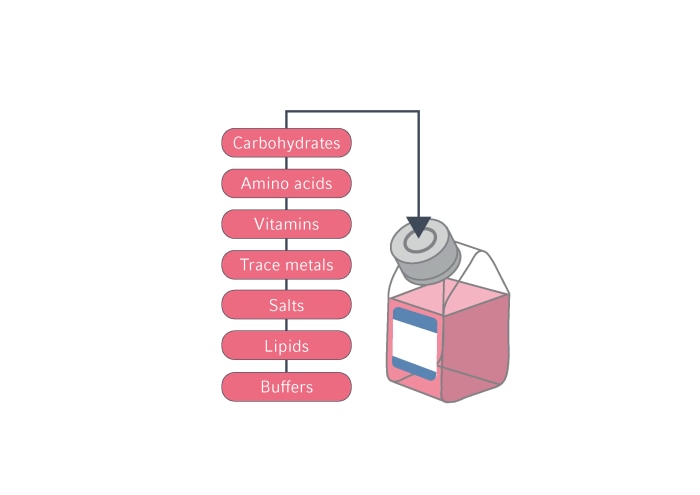
Figure 2. Key components of cell culture media that should be optimized for maximum protein production yields.
Additionally, cell culture components can be used to alleviate stress responses and avoid negative effects on cell growth and productivity. This includes the use of antioxidant compounds, including thiol compounds, α keto acids, vitamins, chelators, phenolic compounds, and trace elements, which reduce oxidative stress in CHO cell systems to increase cell fitness, and ensure high product yield and quality [20].
Read more
Read less
Culture parameters
Process parameters such as temperature, pH, and the partial pressure of carbon dioxide and dissolved oxygen, can have enormous effects on cell viability, metabolism, and product yield and quality. It’s essential to optimize every aspect of your process parameters to enhance the production of recombinant proteins, including:- Temperature shifts from 37 °C to 30–35 °C at 48 hours post-inoculation can extend culture longevity by manipulating the cell cycle. This has been shown to improve production rates and product quality in both CHO and HEK293 cells [21-23]. Bioreactors are designed to modulate temperature within each single bioreactor vessel. For shake flask cultures, expression protocol manufacturers advise that a secondary CO2 incubator that is set at a lower temperature is used, instead of slowly ramping down the temperature.
- It is important to avoid evaporation from cell culture vessels to maintain constant levels of salts, nutrients, and other cell culture components. A high relative humidity of 85 95 % will prevent the evaporation of growth media. Evaporation rates in a bioreactor can also be minimized by exhaust condensation. CO2 incubator shakers with either active humidification or passive water-tray based humidification provide protection against evaporation.
- Dissolved oxygen (DO) concentration is one of the most critical parameters when designing your cell culture, with an optimal DO concentration preventing growth limitations and cytotoxicity. DO is typically within the range of 35-50 % in bioreactor culture. Whereas measurement of DO is also possible in a shake flask [24], DO control is usually not possible.
- Maintaining precise pH control is critical due to its profound influence on cell growth, metabolism, and osmolality. For mammalian cells, like CHO cells, a typical pH target hovers around 7.2 and is regulated through liquid and gas additions in each single bioreactor culture. CO2 is commonly introduced into the medium to lower the pH, while an alkaline solution, such as sodium bicarbonate, is added to elevate the pH. In shake culture, the CO2 concentration is typically controlled indirectly by using CO2 incubator shakers. The chamber atmosphere is usually kept at an atmospheric CO2 concentration between 5 and 8 %, with the optimal concentration depending on the contained buffer system used in the cell culture media.
Read more
Read less
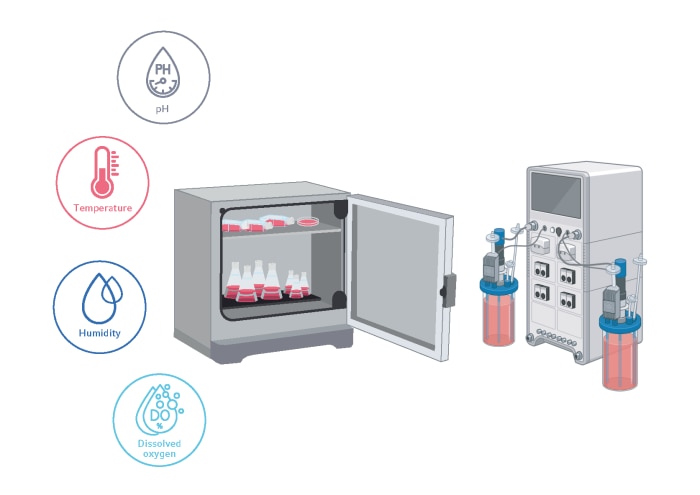
Figure 3. Cell culture conditions that influence cell fitness and protein production yields.
Conclusions and future directions
It is an exciting time for protein production, with increasingly complex proteins and assemblies able to be produced at greater yields and with ever-decreasing timescales. Despite some shortcomings, the production of recombinant proteins via mammalian cell culture is becoming a more routine, rapid, and successful route for both academic and pharmaceutical industries.Overall, there are many effective paths for maximizing protein production in mammalian cells, from gene and vector optimization to cell culture strategies. The key lies in the integration and careful management of these strategies, ensuring high gene expression without compromising cellular resources. As the demand for high-yielding, biologically functional recombinant proteins continues to rise, implementing these and other strategies will become increasingly vital.
Read more
Read less
References
1. Tihanyi, B., & Nyitray, L. (2020). Recent advances in CHO cell line development for recombinant protein production. Drug Discovery Today: Technologies, 38, 25–34. https://doi.org/10.1016/j.ddtec.2021.02.003
2. Chung, B. K.-S., Yusufi, F. N. K., Mariati, Yang, Y., & Lee, D.-Y. (2013). Enhanced expression of codon optimized interferon gamma in CHO cells. Journal of Biotechnology, 167(3), 326–333. https://doi.org/10.1016/j.jbiotec.2013.07.011
3. Shayesteh, M., Ghasemi, F., Tabandeh, F., Yakhchali, B., & Shakibaie, M. (2020). Design, construction, and expression of recombinant human interferon beta gene in CHO-s cell line using EBV-based expression system. Research in Pharmaceutical Sciences, 15(2), 144–153. https://doi.org/10.4103/1735-5362.283814
4. Kim, C. H., Oh, Y., & Lee, T. H. (1997). Codon optimization for high-level expression of human erythropoietin (EPO) in mammalian cells. Gene, 199(1–2), 293–301. https://doi.org/10.1016/s0378-1119(97)00384-3
5. Hia, F., Yang, S. F., Shichino, Y., Yoshinaga, M., Murakawa, Y., et al. (2019). Codon bias confers stability to human mRNA s. EMBO reports, 20(11), e48220. https://doi.org/10.15252/embr.201948220
6. Walsh, I.M., Bowman, M.A., Soto Santarriaga, I.F., Rodriguez, A. and Clark, P.L. (2020). Synonymous codon substitutions perturb cotranslational protein folding in vivo and impair cell fitness. Proceedings of the National Academy of Sciences, 117(7), 3528- 3534. https://doi.org/10.1073/pnas.1907126117
7. Rodova, M., Jayini, R., Singasani, R., Chipps, E., & Islam, M. R. (2013). CMV promoter is repressed by p53 and activated by JNK pathway. Plasmid, 69(3), 223. https://doi.org/10.1016/j.plasmid.2013.01.004
8. Romanova, N., & Noll, T. (2018). Engineered and Natural Promoters and Chromatin-Modifying Elements for Recombinant Protein Expression in CHO Cells. Biotechnology Journal, 13(3), 1700232. https://doi.org/10.1002/biot.201700232
9. Tossolini, I., Gugliotta, A., López Díaz, F., Kratje, R., & Prieto, C. (2022). Screening of CHO-K1 endogenous promoters for expressing recombinant proteins in mammalian cell cultures. Plasmid, 119–120, 102620. https://doi.org/10.1016/j.plasmid.2022.102620
10. Daramola, O., Stevenson, J., Dean, G., Hatton, D., Pettman, G., et al. (2014). A highyielding CHO transient system: Coexpression of genes encoding EBNA-1 and GS enhances transient protein expression. Biotechnology Progress, 30(1), 132–141. https://doi.org/10.1002/btpr.1809
11. Öhman, J., Jakobsson, E., Källström, U., Elmblad, A., Ansari, A., et al. (2006). Production of a truncated soluble human semicarbazide-sensitive amine oxidase mediated by a GST-fusion protein secreted from HEK293 cells. Protein Expression and Purification, 46(2), 321–331. https://doi.org/10.1016/j.pep.2005.10.027
12. Debeljak, N., Feldman, L., Davis, K. L., Komel, R., & Sytkowski, A. J. (2006). Variability in the Immunodetection of His-tagged Recombinant Proteins. Analytical Biochemistry, 359(2), 216–223. https://doi.org/10.1016/j.ab.2006.09.017
13. Reuten, R., Nikodemus, D., Oliveira, M. B., Patel, T. R., Brachvogel, B., et al. (2016). Maltose-Binding Protein (MBP), a Secretion-Enhancing Tag for Mammalian Protein Expression Systems. PloS One, 11(3), e0152386. https://doi.org/10.1371/journal.pone.0152386
14. Ho, S. C. L., Wang, T., Song, Z., & Yang, Y. (2015). IgG Aggregation Mechanism for CHO Cell Lines Expressing Excess Heavy Chains. Molecular Biotechnology, 57(7), 625–634. https://doi.org/10.1007/s12033-015-9852-7
15. Zhou, Y., Raju, R., Alves, C., & Gilbert, A. (2018). Debottlenecking protein secretion and reducing protein aggregation in the cellular host. Current Opinion in Biotechnology, 53, 151–157. https://doi.org/10.1016/j.copbio.2018.01.007
16. Li, Z.-M., Fan, Z.-L., Wang, X.-Y., & Wang, T.-Y. (2022). Factors Affecting the Expression of Recombinant Protein and Improvement Strategies in Chinese Hamster Ovary Cells. Frontiers in Bioengineering and Biotechnology, 10. https://doi.org/10.3389/fbioe.2022.880155
17. McGillicuddy, N., Floris, P., Albrecht, S., & Bones, J. (2018). Examining the sources of variability in cell culture media used for biopharmaceutical production. Biotechnology Letters, 40(1), 5–21. https://doi.org/10.1007/s10529-017-2437-8
18. Ling, W. L. (2015). Development of protein-free medium for therapeutic protein production in mammalian cells: Recent advances and perspectives. PharmaceuticalBioprocessing, 3(3), 215–226. https://doi.org/10.4155/pbp.15.8
19. Li, W., Fan, Z., Lin, Y., & Wang, T.-Y. (2021). Serum-Free Medium for Recombinant Protein Expression in Chinese Hamster Ovary Cells. Frontiers in Bioengineering andBiotechnology, 9. https://www.frontiersin.org/articles/10.3389/fbioe.2021.646363
20. Chevallier, V., Andersen, M. R., & Malphettes, L. (2020). Oxidative stress-alleviating strategies to improve recombinant protein production in CHO cells. Biotechnology andBioengineering, 117(4), 1172–1186. https://doi.org/10.1002/bit.27247
21. Han, Y. K., Koo, T. Y., & Lee, G. M. (2009). Enhanced interferon-beta production by CHO cells through elevated osmolality and reduced culture temperature. Biotechnology Progress, 25(5), 1440–1447. https://doi.org/10.1002/btpr.234
22. Torres, M., Zúñiga, R., Gutierrez, M., Vergara, M., Collazo, N., et al. (2018). Mild hypothermia upregulates myc and xbp1s expression and improves anti-TNFα production in CHO cells. PloS One, 13(3), e0194510. https://doi.org/10.1371/journal.pone.0194510
23. Lin, C.-Y., Huang, Z., Wen, W., Wu, A., Wang, C., et al. (2015). Enhancing Protein Expression in HEK-293 Cells by Lowering Culture Temperature. PLOS ONE, 10(4), e0123562. https://doi.org/10.1371/journal.pone.0123562
24. Maschke, R. W., Pretzner, B., John, G. T., Herwig, C., & Eibl, D. (2022). Improved Time Resolved KPI and Strain Characterization of Multiple Hosts in Shake Flasks Using Advanced Online Analytics and Data Science. Bioengineering (Basel, Switzerland), 9(8), 339. https://doi.org/10.3390/bioengineering9080339
Read more
Read less
Related Links
Read more
Read less