MENU
IE | EUR
IE | EUR
You are about to leave this site.
Please be aware that your current cart is not saved yet and cannot be restored on the new site nor when you come back. If you want to save your cart please login in into your account.
myEppendorf
No results found
Search Suggestions
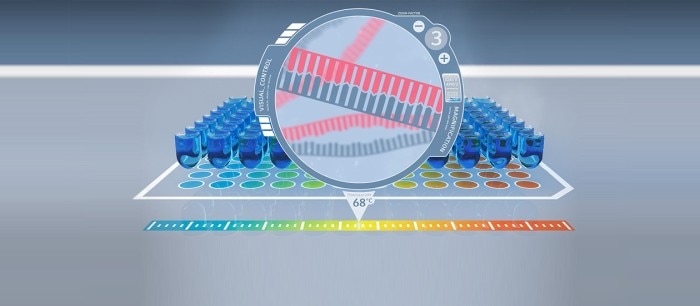
Gradient PCR for Optimization
Lab Academy
- Molecular Biology
- PCR Cyclers
- Amplification & PCR
- Essay
Anyone who has performed PCR would be familiar with the three basic steps in the “chain reaction” – denaturation, annealing and extension. In general, the temperature used in denaturation step is dependent on the DNA
The extension temperature is generally set at 72°C because Taq polymerase works optimally at this temperature. This means at each step, there is a degree of flexibility in the temperature.
The positive consequence of this is versatility – temperatures can be modified to suit almost any applications, steps can be combined to shorten the total reaction time, multiple PCRs can be run together using a shared condition, etc. However, the problem with any versatility is, finding the “best” condition becomes almost an art. If everything is possible, finding “the one” is like searching for a single fish in an ocean. Fortunately, there are some guidelines to follow and it is not to the point of despair.
For example, we know that the denaturation temperature should be as low as reasonable to denature template DNA and thus allowing efficient primer annealing and strand extension1 . At the same time, it should not be too high to prevent loss of enzyme activity since half-life of Taq DNA polymerases drastically reduce with increasing temperature2 . Naturally, decades of improvements see more and more stable PCR reagent formulations – increasing fidelity, specificity and speed performance of enzymes. Thus, the denaturation temperature generally falls between 92°C to 98°C, offering choices based on users’ applications. For example, difficult templates such as GC-rich sequences require higher temperature. Higher denaturation temperature can also lead to higher specificity in PCR amplification3 .
Things become more complicated when choosing the right annealing temperature. The closest reference one has is the melting temperatures (TM) of the forward and reverse primers. TM describe the temperature at which there is an equilibrium between primer bound to DNA and primer dissociating from DNA, resulting in 50% of the template having bound primer. When choosing primer pairs, it is important that the TM of both primers not differ from each other by more than 5°C. After all, you must choose only one temperature to perform the annealing with. The bigger the difference, the more bias you have towards one primer or the other.
Unfortunately, TM can change with reagent concentration, pH and salt concentration. Hence, TM remains a theoretical value that can only provide a rough guideline to determine the actual annealing temperature (TA). Using higher TA results in higher specificity but may affect the yield as it means less binding (more stringent binding condition). Thus, finding the most efficient and specific TA is often a trial and error endeavor. This was the main driver behind the innovation of “gradient technology”.
What is gradient technology? It is a technology where instead of giving the same temperature throughout the entire thermal block of a thermal cycler, each of the columns or rows in the block is made to have different temperatures. This means that you can either choose to run repeated PCRs testing different temperatures – each about an hour long – to find the right TA. Or, you can choose to use a thermal cycler equipped with gradient function. With it, you can run different temperatures all at once, saving you much time and effort.
The positive consequence of this is versatility – temperatures can be modified to suit almost any applications, steps can be combined to shorten the total reaction time, multiple PCRs can be run together using a shared condition, etc. However, the problem with any versatility is, finding the “best” condition becomes almost an art. If everything is possible, finding “the one” is like searching for a single fish in an ocean. Fortunately, there are some guidelines to follow and it is not to the point of despair.
For example, we know that the denaturation temperature should be as low as reasonable to denature template DNA and thus allowing efficient primer annealing and strand extension1 . At the same time, it should not be too high to prevent loss of enzyme activity since half-life of Taq DNA polymerases drastically reduce with increasing temperature2 . Naturally, decades of improvements see more and more stable PCR reagent formulations – increasing fidelity, specificity and speed performance of enzymes. Thus, the denaturation temperature generally falls between 92°C to 98°C, offering choices based on users’ applications. For example, difficult templates such as GC-rich sequences require higher temperature. Higher denaturation temperature can also lead to higher specificity in PCR amplification3 .
Things become more complicated when choosing the right annealing temperature. The closest reference one has is the melting temperatures (TM) of the forward and reverse primers. TM describe the temperature at which there is an equilibrium between primer bound to DNA and primer dissociating from DNA, resulting in 50% of the template having bound primer. When choosing primer pairs, it is important that the TM of both primers not differ from each other by more than 5°C. After all, you must choose only one temperature to perform the annealing with. The bigger the difference, the more bias you have towards one primer or the other.
Unfortunately, TM can change with reagent concentration, pH and salt concentration. Hence, TM remains a theoretical value that can only provide a rough guideline to determine the actual annealing temperature (TA). Using higher TA results in higher specificity but may affect the yield as it means less binding (more stringent binding condition). Thus, finding the most efficient and specific TA is often a trial and error endeavor. This was the main driver behind the innovation of “gradient technology”.
What is gradient technology? It is a technology where instead of giving the same temperature throughout the entire thermal block of a thermal cycler, each of the columns or rows in the block is made to have different temperatures. This means that you can either choose to run repeated PCRs testing different temperatures – each about an hour long – to find the right TA. Or, you can choose to use a thermal cycler equipped with gradient function. With it, you can run different temperatures all at once, saving you much time and effort.
Read more
Read less
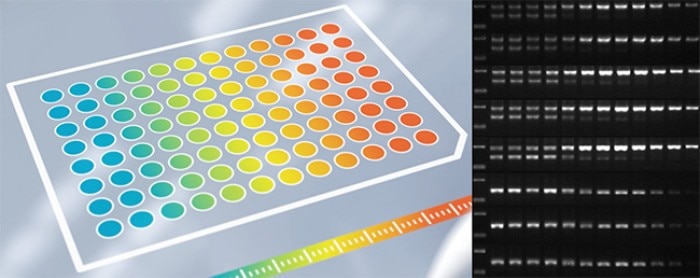
Based on the analysis step, e.g. gel electrophoresis, the best annealing temperature can be defined. Of course, the higher the number of temperatures you can run at one go, the more you know about your primer behaviour, making future optimization work easier, resulting in potential savings in time and money.
References:
[1] McPherson, M. & Moller, S. (2006) PCR 2nd edition (https://saddam2014.files.wordpress.com/2014/01/pcr-taylor-francis-2006.pdf)
[2] Innis, M., & Gelfand, D. H. (1999) Optimization of PCRs (http://www.ispybio.com/search/protocols/PCR%20protocol9.pdf)
[3] Gerke, N. & Hellberg, A. (2013) Eppendorf Application Note 289
References:
[1] McPherson, M. & Moller, S. (2006) PCR 2nd edition (https://saddam2014.files.wordpress.com/2014/01/pcr-taylor-francis-2006.pdf)
[2] Innis, M., & Gelfand, D. H. (1999) Optimization of PCRs (http://www.ispybio.com/search/protocols/PCR%20protocol9.pdf)
[3] Gerke, N. & Hellberg, A. (2013) Eppendorf Application Note 289
Read more
Read less