メニュー
JP | JPY
-
-
-
- Challenges and Chances: A Review of the 1st Stem Cell Community Day
- Summertime, and the Livin’ Is Easy…
- Follow-on-Biologics – More than Simple Generics
- Bacteria Versus Body Cells: A 1:1 Tie
- Behind the Crime Scene: How Biological Traces Can Help to Convict Offenders
- Every 3 Seconds Someone in the World Is Affected by Alzheimer's
- HIV – It’s Still Not Under Control…
- How Many Will Be Convicted This Time?
- Malaria – the Battle is Not Lost
- Physicians on Standby: The Annual Flu Season Can Be Serious
- At the Forefront in Fighting Cancer
- Molecular Motors: Think Small and yet Smaller Again…
- Liquid Biopsy: Novel Methods May Ease Cancer Detection and Therapy
- They Are Invisible, Sneaky and Disgusting – But Today It’s Their Special Day!
- How Many Cells Are in Your Body? Probably More Than You Think!
- What You Need to Know about Antibiotic Resistance – Findings, Facts and Good Intentions
- Why Do Old Men Have Big Ears?
- The Condemned Live Longer: A Potential Paradigm Shift in Genetics
- From Research to Commerce
- Chronobiology – How the Cold Seasons Influence Our Biorhythms
- Taskforce Microbots: Targeted Treatment from Inside the Body
- Eyes on Cancer Therapy
-
-
-
-
- Challenges and Chances: A Review of the 1st Stem Cell Community Day
- Summertime, and the Livin’ Is Easy…
- Follow-on-Biologics – More than Simple Generics
- Bacteria Versus Body Cells: A 1:1 Tie
- Behind the Crime Scene: How Biological Traces Can Help to Convict Offenders
- Every 3 Seconds Someone in the World Is Affected by Alzheimer's
- HIV – It’s Still Not Under Control…
- How Many Will Be Convicted This Time?
- Malaria – the Battle is Not Lost
- Physicians on Standby: The Annual Flu Season Can Be Serious
- At the Forefront in Fighting Cancer
- Molecular Motors: Think Small and yet Smaller Again…
- Liquid Biopsy: Novel Methods May Ease Cancer Detection and Therapy
- They Are Invisible, Sneaky and Disgusting – But Today It’s Their Special Day!
- How Many Cells Are in Your Body? Probably More Than You Think!
- What You Need to Know about Antibiotic Resistance – Findings, Facts and Good Intentions
- Why Do Old Men Have Big Ears?
- The Condemned Live Longer: A Potential Paradigm Shift in Genetics
- From Research to Commerce
- Chronobiology – How the Cold Seasons Influence Our Biorhythms
- Taskforce Microbots: Targeted Treatment from Inside the Body
- Eyes on Cancer Therapy
-
JP | JPY
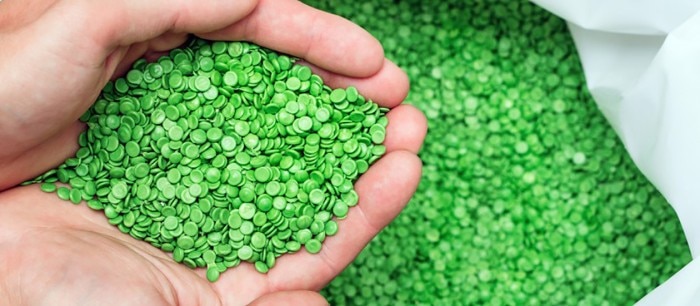
Bioplastic in the Lab?
Lab Academy
- サステナビリティ
- 細胞培養用消耗品
- エッセー
Bioplastics are often discussed as a more sustainable, environmentally friendly alternative to conventional plastics thereby reducing our reliance on fossil fuels. Here, we look at bioplastics and their applications in the lab – what are bioplastics, how do they work, and can they help labs become more sustainable in the future?
Manufacturers of lab consumables are constantly looking to improve their product range to satisfy ever-changing customer demands while continuing to deliver the highest level of purity, quality, and consistency. These improvements have led – and are continuing to lead – to new products for emerging applications and improved versions of existing products.Increasingly, lab managers and scientists are seeking to improve sustainability in the lab. For some this is a key priority and obviously the amount and type of plastics used plays an important role in this. Since bioplastics use biomass from renewable sources – rather than from fossil fuels – for polymer production, could switching to bioplastic-based consumables improve lab sustainability?
Bioplastics are also known as plastics from renewable biomass. Instead of using oil-based polymer building blocks, bioplastics use processed biomass (for example bioethanol, starch, cellulose, or lactic acid) as the starting material – thereby reducing reliance on oil. Bioplastics are easily confused with bio-degradable plastics, however not all bioplastics are biodegradable. You can find out more about biodegradable plastics here 1,2 .
Although the range of polymers where at least a small percentage of the total production uses renewable biomass is small compared to the range of polymers made from oil derivatives, there are many well-known plastics that now have a bioplastic alternative. For example, the most commonly used plastic worldwide, polyethylene, can be produced from bioethanol. Other common polymers that can be made from renewable biomass are polylactic acid (PLA), polyhydroxyalkanoates (PHAs), cellulose acetate, and nitrocellulose1 .
The raw materials needed for production of bioplastics can come from different natural sources depending on the type of polymer produced and the production process. Some processes use waste materials or non-edible parts of plants, but the majority requires edible crops. Processes that use edible crops are often more efficient, but have the disadvantage of competing with food production1 .
Polymers used for the production of lab consumables, such as polypropylene and polystyrene, are currently not made from renewable biomass, however in this rapidly developing industry this may become possible in the near future3 . For this reason, it is important to look closely at the positive and negative effects of bioplastics on the environment1,3,4 .
One clear benefit is that bioplastics do not require as many oil-based products in their production, because the polymer building blocks are, at least in part, plant-derived. As a result, bioplastics have a reduced carbon footprint and are associated with lower greenhouse gas emissions during production1,3,4 .
A key drawback to the production of bioplastics is the sourcing of raw materials. Bioderived polyethylene, for example, is usually produced from high-sugar or high-starch crops, which require large amounts of energy and arable land to grow the quantities needed for large-scale bioplastic production. As a result, its production competes with food or animal feed production, which can influence supply2,5 .
Using modern large-scale agriculture practices for plastics production also has an impact on soil and water quality. This primarily relates to the acidification and eutrophication (i.e. an ecosystem becoming overly enriched with nutrients, such as phosphorus and nitrogen) of surface water – generally the result of overuse of fertilizers. Acidification and eutrophication have a negative effect on the quality of an ecosystem as they cause, for example, rapid growth of algae, which may cause toxicity and loss of biodiversity1 .
For the reasons mentioned above, academics and governmental organizations have raised doubts about whether bioplastics have a clear positive effect on the environment. For example, the German federal environmental agency (UBA) stated that manufacturers have not proved that their bioplastic products offer at least equivalent environmental sustainability compared to products made from traditional plastics6,7 .
A recent study from the University of Bonn also investigated the impact of increased bioplastic production and concluded that setting, for example, a 5% target for bioplastic consumption by using food crops would not be an effective strategy for climate change mitigation5.
The range of bioplastics is continuously expanding and, in the future, it may be possible to produce bioplastics that fulfill all requirements of lab users in terms of purity, quality, and consistency. If this becomes a possibility, it will be important to look closely at all environmental aspects of the bioplastic production process and the sourcing of raw materials. As the overall environmental impact of many current methods is debatable, improvements in production methods will hold the key to answering the question whether bioplastics will one day become a viable alternative for lab consumables.
1. Cho, R. The truth about bioplastics. 2017. https://phys.org/news/2017-12-truth-bioplastics.html
2. Bušić, A et al. Bioethanol Production from Renewable Raw Materials and Its Separation and Purification: A Review. Food Technology and Biotechnology 2018;56(3): 289–311.
3. Thryft AR. Bioplastics Get Tougher and Greener. Design News 2018. https://www.designnews.com/materials-assembly/bioplastics-get-tougher-and-greener/152608211258669
4. Posen D et al. Greenhouse gas mitigation for U.S. plastics production: energy first, feedstocks later. Environmental Research Letters 2017;12:3.
5. Escobar, N et al. Land use mediated GHG emissions and spillovers from increased consumption of bioplastics. Environmental Research Letters 2018;13.
6. Beier W. Biologisch Abbaubare Kunststoffe. Umweltbundesamt 2009. https://www.umweltbundesamt.de/sites/default/files/medien/publikation/long/3834.pdf
7. No author. Kurzposition Biokunststoffe. Umweltbundesamt 2017. https://www.umweltbundesamt.de/sites/default/files/medien/2503/dokumente/uba_kurzposition_biokunststoffe.pdf.
What makes a plastic a bioplastic?
Bioplastics are also known as plastics from renewable biomass. Instead of using oil-based polymer building blocks, bioplastics use processed biomass (for example bioethanol, starch, cellulose, or lactic acid) as the starting material – thereby reducing reliance on oil. Bioplastics are easily confused with bio-degradable plastics, however not all bioplastics are biodegradable. You can find out more about biodegradable plastics here 1,2 . Although the range of polymers where at least a small percentage of the total production uses renewable biomass is small compared to the range of polymers made from oil derivatives, there are many well-known plastics that now have a bioplastic alternative. For example, the most commonly used plastic worldwide, polyethylene, can be produced from bioethanol. Other common polymers that can be made from renewable biomass are polylactic acid (PLA), polyhydroxyalkanoates (PHAs), cellulose acetate, and nitrocellulose1 .
The raw materials needed for production of bioplastics can come from different natural sources depending on the type of polymer produced and the production process. Some processes use waste materials or non-edible parts of plants, but the majority requires edible crops. Processes that use edible crops are often more efficient, but have the disadvantage of competing with food production1 .
The bioplastics sustainability paradox
Polymers used for the production of lab consumables, such as polypropylene and polystyrene, are currently not made from renewable biomass, however in this rapidly developing industry this may become possible in the near future3 . For this reason, it is important to look closely at the positive and negative effects of bioplastics on the environment1,3,4 . One clear benefit is that bioplastics do not require as many oil-based products in their production, because the polymer building blocks are, at least in part, plant-derived. As a result, bioplastics have a reduced carbon footprint and are associated with lower greenhouse gas emissions during production1,3,4 .
A key drawback to the production of bioplastics is the sourcing of raw materials. Bioderived polyethylene, for example, is usually produced from high-sugar or high-starch crops, which require large amounts of energy and arable land to grow the quantities needed for large-scale bioplastic production. As a result, its production competes with food or animal feed production, which can influence supply2,5 .
Using modern large-scale agriculture practices for plastics production also has an impact on soil and water quality. This primarily relates to the acidification and eutrophication (i.e. an ecosystem becoming overly enriched with nutrients, such as phosphorus and nitrogen) of surface water – generally the result of overuse of fertilizers. Acidification and eutrophication have a negative effect on the quality of an ecosystem as they cause, for example, rapid growth of algae, which may cause toxicity and loss of biodiversity1 .
For the reasons mentioned above, academics and governmental organizations have raised doubts about whether bioplastics have a clear positive effect on the environment. For example, the German federal environmental agency (UBA) stated that manufacturers have not proved that their bioplastic products offer at least equivalent environmental sustainability compared to products made from traditional plastics6,7 .
A recent study from the University of Bonn also investigated the impact of increased bioplastic production and concluded that setting, for example, a 5% target for bioplastic consumption by using food crops would not be an effective strategy for climate change mitigation5.
Bioplastics moving forward
The range of bioplastics is continuously expanding and, in the future, it may be possible to produce bioplastics that fulfill all requirements of lab users in terms of purity, quality, and consistency. If this becomes a possibility, it will be important to look closely at all environmental aspects of the bioplastic production process and the sourcing of raw materials. As the overall environmental impact of many current methods is debatable, improvements in production methods will hold the key to answering the question whether bioplastics will one day become a viable alternative for lab consumables.
References
1. Cho, R. The truth about bioplastics. 2017. https://phys.org/news/2017-12-truth-bioplastics.html 2. Bušić, A et al. Bioethanol Production from Renewable Raw Materials and Its Separation and Purification: A Review. Food Technology and Biotechnology 2018;56(3): 289–311.
3. Thryft AR. Bioplastics Get Tougher and Greener. Design News 2018. https://www.designnews.com/materials-assembly/bioplastics-get-tougher-and-greener/152608211258669
4. Posen D et al. Greenhouse gas mitigation for U.S. plastics production: energy first, feedstocks later. Environmental Research Letters 2017;12:3.
5. Escobar, N et al. Land use mediated GHG emissions and spillovers from increased consumption of bioplastics. Environmental Research Letters 2018;13.
6. Beier W. Biologisch Abbaubare Kunststoffe. Umweltbundesamt 2009. https://www.umweltbundesamt.de/sites/default/files/medien/publikation/long/3834.pdf
7. No author. Kurzposition Biokunststoffe. Umweltbundesamt 2017. https://www.umweltbundesamt.de/sites/default/files/medien/2503/dokumente/uba_kurzposition_biokunststoffe.pdf.
もっと読む
表示を減らす